Biomedical Imaging
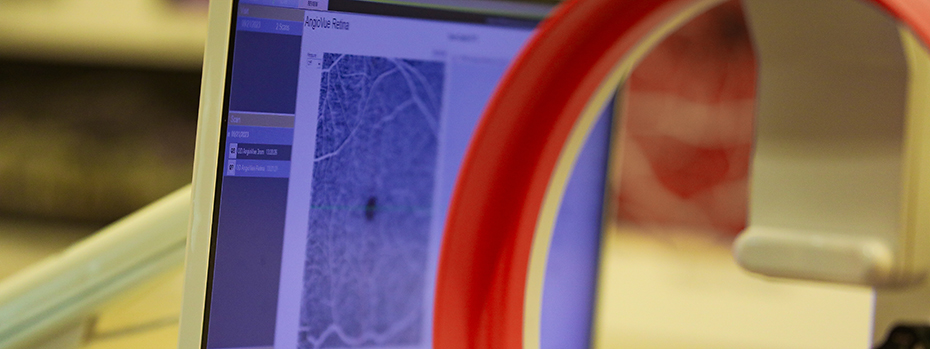
At OHSU, biomedical imaging researchers develop new ways to see inside the body, visualize disease processes and analyze data and images. Our faculty have pioneered imaging technology in ophthalmology, super resolution single-molecule microscopy and the use of fluorescent probes to protect nerves during surgery.
Researchers work closely with the Casey Eye Institute and the Knight Cancer Institute to improve early detection and treatment of various eye disorders and cancer.
Our projects include:
- Developing image analysis tools to quantify multiscale imaging and gain insights into the spatial distribution of cancer cells and their interactions within the tumor microenvironment. (Chang Lab)
- Developing optimized imaging reagents to expand the capabilities of patient-specific imaging. (Gibbs Lab)
- Design and develop contrast agents to improve surgery, with a focus on nerve-specific fluorescent agents to aid in nerve identification, visualization and sparing during an operation. (Gibbs Lab)
- Study of immune cell trafficking in the iris of the eye. (Yali Jia Lab)
- Lanthanide-loaded nanoparticles as potential fluorescent and mass probes for high-content protein analysis and monitoring. (Yantasee Lab)
- Imaging cancer patient blood cells at the molecular level to help a person track the success of their treatment prior to, during and following cancer therapy. (Vu Lab)
- Development of a pointillist microscopy system to visualize and quantify the formation of molecular aggregates (condensates). (Galbraith Lab)
- Advanced microscopy techniques (single-molecule, light sheet, novel fluorescent dyes) to understand molecular mechanisms underlying migration in normal and diseased cells. (Galbraith Lab)
Join our program
Your research career starts here.