Cardiovascular Engineering
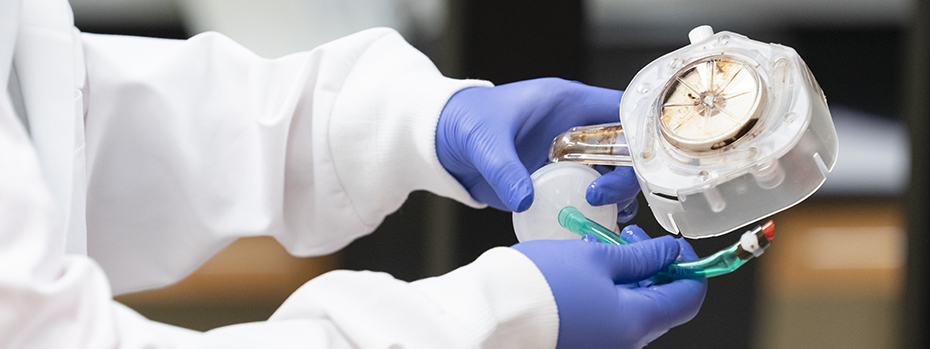
Cardiovascular disease is the leading cause of death in the United States. As a student in our cardiovascular labs, you will help us discover new strategies and devices to diagnose, prevent and treat heart and circulation problems.
By applying engineering principles like fluid mechanics and chemical adhesion, we gain new insights into heart-related biological processes. Already, OHSU research has led to the development of medicines that treat clotting disorders without an increased risk of bleeding.
Cardiovascular researchers at OHSU study:
- Clotting
- Cardiac tissue development
- Biomechanics of the heart and blood vessels
- Cardiovascular tissue regeneration
Our projects include:
- Identification of thrombogenic and inflammatory triggers, including those that are associated with metastatic cancer, infections and injuries, to develop new molecular targets for treatment. (McCarty Lab)
- Exploring the origins of congenital heart disease, particularly the role of blood flow dynamics on embryonic heart development. (Rugonyi Lab)
- Understanding hemodynamics and vascular cell functions and designing new devices to treat cardiovascular disease, including vascular stents and grafts, protein-based biomaterials and noninvasive and nondestructive evaluation of engineered tissues. (Hinds Lab)
Join our program
Your research career starts here.