Projects
How do neurons pass information between themselves, thus allowing us to learn and remember, and how do the hair cells of the ear convert mechanical forces into an electrical signal? These are the two areas that we focus on, not only to understand, in structural and biochemical terms, how the nervous systems ‘works’, but also so that we can understand how disease-associated mutations derail function and how new small molecules might be created to modulate function. To illuminate mechanism, we employ a range of structural and biophysical techniques, from single molecule fluorescence to cryo-electron microscopies, in synergy with biochemical, electrophysiological and crystallographic methods. We are particularly excited about bringing electron microscopy methods to bear on these questions, aiming to more fully understand how neurotransmitter receptors, transporter and mechanically-gated ion channels function in their native or native-like environments.
Nearly all aspects of brain development and function, as well as many aspects of disease and injury, directly involve signaling at glutamatergic synapses. Because of the crucial roles that glutamate receptors — AMPA, kainate and NMDA — play in neuronal function, we have fostered a long standing interest in understanding, on the one hand, the molecular details of receptor assembly, gating and modulation while on the other hand, we have an emerging interest in elucidating the architecture of native, heteromeric glutamate receptors and their complexes with auxiliary proteins. Moreover, we are beginning to exploit novel reagents developed in the laboratory to facilitate the identification and imaging of native receptors at physiological synapses using a combination of light and electron microscopies.
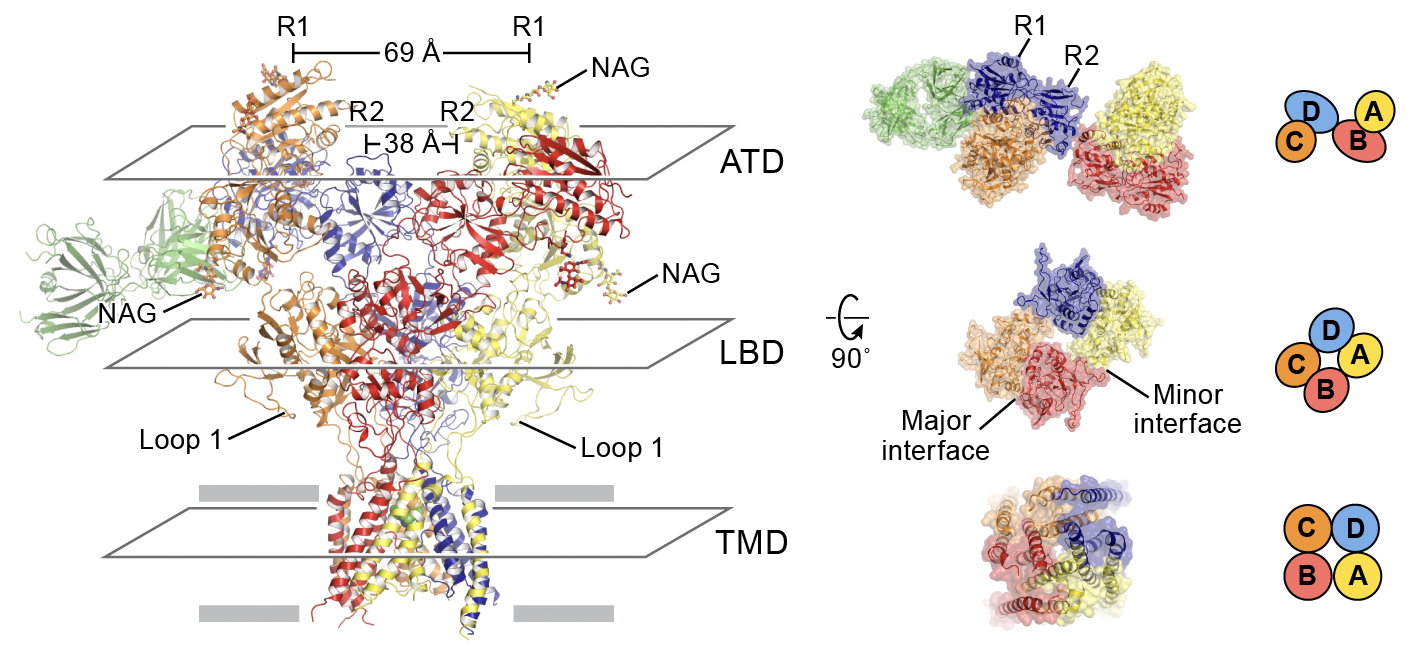
Left panel: Cartoon representation of the model of triheteromeric NMDAR in the non-Ro-bound state, viewed parallel to the membrane plane. Right panel: Structures viewed from the extracellular side of the membrane representing the subunit arrangement in the ATD (top), LBD (middle) and TMD (bottom) layers.
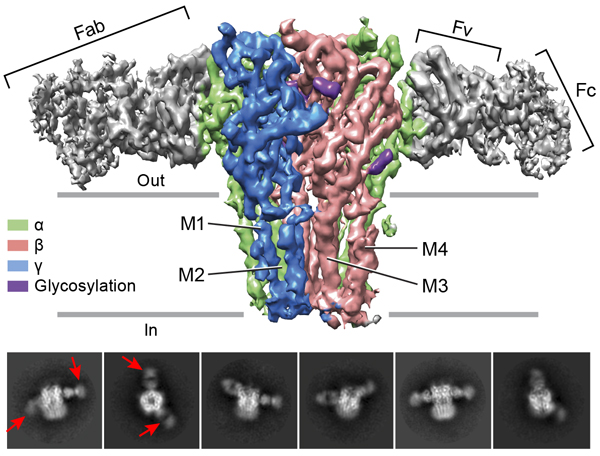
Pentameric Cys-loop receptors, in addition to glutamate receptors, are also fundamental to the development and function of the central and peripheral nervous system and define a second major class of neurotransmitter gated ion channels. Whereas glutamate receptors are cation selective ion channels, we are particularly interested in the classes of Cys-loop receptors that are anion, or chloride selective, in large part because these ion channels provide inhibitory balance or ‘tone’ to neuronal circuits and, as it turns out, are important therapeutic targets and are implicated in a number of genetic diseases. Thus, we want to understand how anesthetic agents as well as sedatives and anticonvulsive medications interact with and modulate the properties of heteromeric GABAA receptors, as well as to define the architecture of GABAA receptor signaling complexes at synapses. The gating of Cys-loop receptors has been studied for decades yet structure-based understanding of gating mechanisms remain unresolved. Thus we have focused on the homomeric glycine receptor as the paradigm by which to elucidate structural principles of gating as well as the action of full and partial agonists. By harnessing the latest developments in single particle cryo-EM, we carry out reconstructions at near atomic resolution, thus not only allowing for visualization of the receptor and agonists, but also of ions within the permeation pathway.
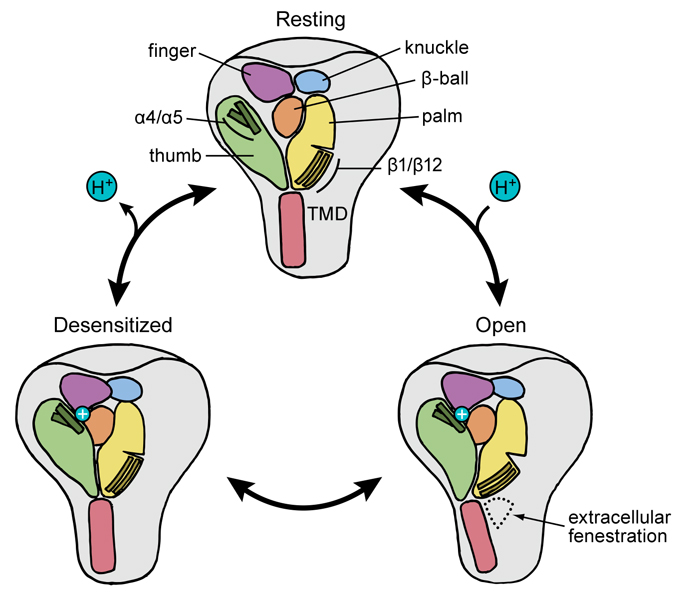
Ion channels create tiny openings in the membrane of cells throughout the body, allowing the transmission of signals in the nervous system. Acid-sensing ion channels are believed to play a role in pain sensation as well as psychiatric disorders. The Gouaux lab recently revealed the atomic structure of an isolated acid-sensing ion channel in a resting state, bringing into focus the full range of motion for the channel, as the desensitized and open states previously had been revealed in research published in 2009 and 2014. Our next phase of this research will involve studying the channels embedded within tissue to better understand how they interact with other key proteins within the cellular membrane.
Signaling at chemical synapses is a ‘ring cycle’ of 3 separable ‘performances’: release, detection and uptake. Whereas receptors, such as the glutamate and Cys-loop receptors mediate detection, it is the transporters that remove transmitter from synaptic spaces, thus quenching the signal and allowing for subsequent cycles of release and detection. Even though we know a great deal about the overall structure of the crucial neurotransmitter transporters for glutamate, glycine, GABA and the biogenic amines (serotonin, dopamine, norepinephrine) from studies of bacterial homologs, we know much less about the eukaryotic transporters and, in particular, how their structures and activities are modulated by allosteric ligands and post-translational modifications. Moreover, the mystery of how drugs of addiction, such as the amphetamines, alter transporter activity and promote transmitter release remains unsolved. With the advances in single particle cryo-EM, together with antibody fragments developed in this laboratory, elucidating high resolution structures by electron microscopy is now within reach and, once realized, will allow one to carry out 3D classification to elucidate all of the major conformations populated throughout the transporter transport cycle.
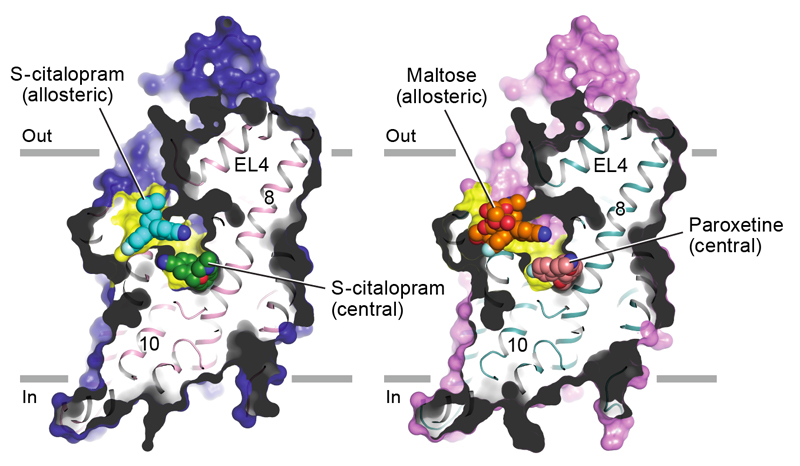
Left panel: Sagittal slice through a surface representation of the (S)-citalopram-bound transporter. (S)-citalopram molecules bound to the allosteric (cyan) and central (green) sites are shown as spheres. Right panel: A maltose head group (orange), derived from a detergent molecule and bound to the allosteric site, and paroxetine (pink), bound to the central site, are shown as spheres.
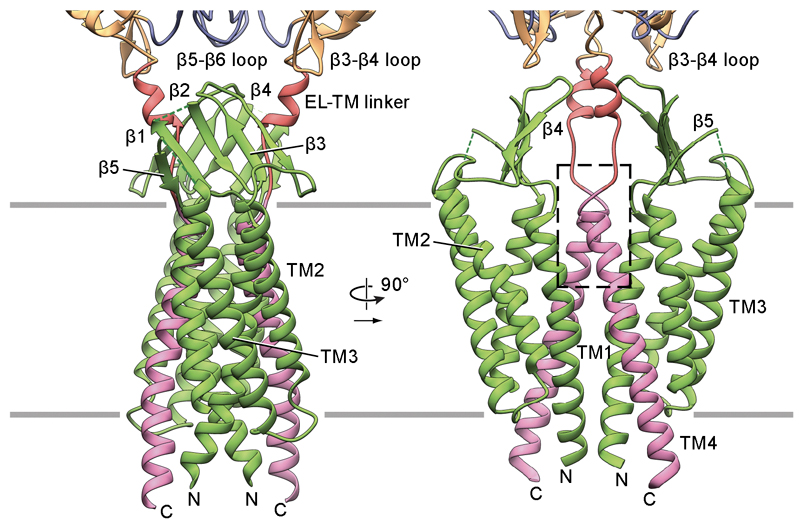
The sensing of sound, movement and balance across the vertebrate kingdom originates within hair cells in the inner ear. In mammals, hair cells within the cochlea mediate our sensation of sound while the detection of movement and our sense of balance originates with hair cells of the utricle and saccule. Hair cells have a characteristic morphology and harbor actin-filled stereocilia ‘staircases’ in which the stereocilia are coupled by protocadherin 15 (PCDH15) molecules from one stereocilium linked with cadherin 23 (CDH23) molecules from an adjacent stereocilium. Upon movement of the stereocilia induced by fluctuations of the surrounding fluid, the PCDH15-CDH23 ‘tip links’ trigger the opening of a mechano-electrical transduction (MET) ion channel at the stereocilia tips. While the molecular composition of the MET channel is enigmatic, the fundamental role of PCDH15 in coupling the movement of the stereocilia to the gating of the MET channel is unambiguous and conserved throughout vertebrates. In humans, mutations in PCDH15 cause Usher syndrome type 1, an inherited deaf-blindness together with vestibular dysfunction and unintelligible speech, as well as nonsyndromic recessive hearing loss (DFNB23).
Our recent studies have illuminated the architecture of the PCDH15-LHFPL5 complex, localized mutations associated with deafness, and shed new light on how forces in the PCDH15 tether may be transduced into the stereocilia membrane.